Quarks - Fundamental Constituents of Matter
As a participant, I've gained a better understanding of certain concepts linked to the #citizenscience project which is presently on-chain here on Hive. This is an initiative of particle physicist @lemouth and this blog is an attempt to explain my novice understanding of quarks in the hopes of assisting other individuals who are like me.
We were taught as children that everything around us is made up of atoms, which are made up of protons, neutrons, and electrons. And, if we were lucky, we might learn what protons and neutrons are comprised of.
It's a good thing we now know that they're each made up of three quarks. But then the question arises: "How are protons and neutrons different if they're both made of quarks?"
To put it another way, protons are considered to be made up of three quarks. However, protons are not as straightforward as that. The proton will always have two upquarks with a charge of (+2/3) apiece and a downquark with a charge of (-1/3), all of which sum up to +1, which is the proton's charge.
-> (2/3) + (2/3) - (1/3) = +1
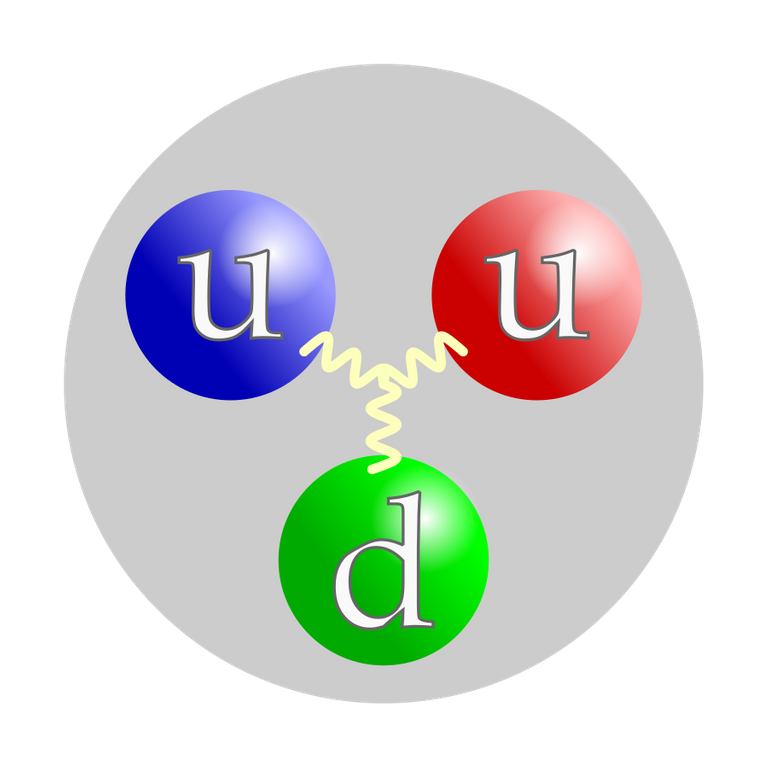
The quark structure of the proton. There are two up quarks in it and one down quark. The strong force is mediated by gluons (wavey); Author- Jacek_rybak; License
These three quarks are referred to as "Valence Quarks," and they are undeniably present. The proton as well may have an extra "upquark-antiupquark" pair.
Wondering what an antiquark is?
Hmmm, it's just a quark's anti-particle.
Other sorts of quarks, such as pairings of "strangequark-antistrangequark," "charmquark-anticharmquark," and so on, could be present in the proton. In reality, the proton is likely to contain a large number of quark-antiquark couples.
Six of the particles in the Standard Model are quarks (shown in purple). Each of the first three columns forms a generation of matter. Source; Public Domain
Please Note that the masses of certain particles are subject to periodic reevaluation by the scientific community. The values currently reflected in this graphic are as of 2019 and may have been adjusted since. For the latest consensus, please visit the Particle Data Group website
Interestingly, the strong force, which is carried by particles called gluons, holds the quarks together. This indicates that there are zillions of gluons and quarks inside the proton, all travelling at close to light speed and smashing with one another. During the collisions, they annihilate themselves, resulting in the formation of new ones. That's the quantum world in a proton; :) More like a wild and chaotic party;)
This leads to the next question: as wouldn't such scenarios break some form of conservation law if particles happen to appear in the proton?
Quarks, however, are capable of doing so. They can appear and vanish, but not out of nothing. According to Einstein's famous equation "E = mc2", mass can be converted into a large amount of energy, or a large amount of energy can be converted into mass. In actuality, the quarks that make up a proton account for only 1% of the proton's total mass. It's like putting three coins in a sack and having it suddenly weigh ten pounds.
There's a lot of energy in the gluon field, and there's a lot of energy in the movement of those fast particles. As "quark-antiquark" pairs pop in and out of existence, all of this energy contributes to the mass of the proton, and all we need to conserve is the proton's total mass energy.
There are a few other constraints though. Such as; the total number of upquarks must be two more than the total number of anti-upquarks. Also, one more downquark must exist than anti-downquark. This brings the total number of valence quarks to three, and the quark-antiquark pairs of other types must cancel out. These include; the topquark, downquark, strangequark, and bottomquark.
Other features like charge must be retained when quark-pairs appear spontaneously in the proton. As a result, if a quark with a charge of (+2/3) appears, its partner must have a charge of (-2/3). Similarly, the particles' spins must be opposite, and the proton's colorlessness must be preserved.
It's worth noting that quarks all have color. However, it's not the same as real color. Color in quarks is a form of charge comparable to an electric charge, although it corresponds to the strong force rather than the electromagnetic force.
Quarks, like the three fundamental hues of light, can be red, blue, or green. We get white light when we mix those colors. As a result, when we say the protons are colorless, we're implying that their three valence quarks are red, blue, and green.
The color of the quarks can change, but the overall color must remain white. Antiquarks have the opposite color to quarks, therefore they can be thought of as a "blue-antiblue" pair, with anti-blue cancels out blue!
To me, the most astonishing thing about quarks is that scientists know all there is to know about them without ever ever detecting one. Isn't it fascinating?! In fact, detecting just one is difficult because they are never seen alone. The more quarks scientists try to separate, the more energy they must expend to tear them apart. They finally expend enough energy to create the mass of two new quarks, which can then be bound to the original two.
So, in summary, there are three valence quarks and an infinite number of additional quarks. And I'm hoping that this will help us better comprehend quarks when we come across them in the # citizenscience project! On his blog, @lemouth has a series of posts about these particles and more. Thank you for taking the time to read this.
For more you can check out these links:
Charmonia, bottomonia & toponia - the fantastic beasts of particle physics
Antiquark
Quark
Extreme State of Matter: Evidence of Top Quarks in Collisions at the Large Hadron Collider
Top quark physics at hadron colliders
Posted from HypeTurf
Thanks for this very nice blog and for sharing it with us! It is always a pleasure to read about particle physics from non-particle-physicist authors.
To rephrase a bit what you wrote, protons are indeed not “simply” made of three quarks. Protons are dynamic objects in which we have continuous interactions between quarks, antiquarks and gluons. Quarks can emit gluons, and gluons can convert themselves in a quark-antiquark pair (of any flavour) or into a pair of gluons. The proton’s content is thus much richer than what we could naively think about, and the dynamic behaviour moreover depends on the energy (accelerated protons in the LHC do not behave as protons at rest).
If you remember the tasks of the second episode of our citizen science project, the proton was defined in MG5aMC as
This means that we have up quarks, down quarks, strange quarks, charm quarks, all corresponding antiquarks and gluons inside the proton. We then have parton distribution functions indicating us how to relate the proton itself to its content. This is a bit what I mentioned here.
Finally, I will object one of the points raised in your blog:
This is incorrect. The top quark (that is special by virtue of its large mass) has been detected alone (although through its decay products). Yeah, the top quark is a weird beast ;)
Cheers!
PS: you may be interested in this older blog of mine on quark-antiquark bound states.
Thanks for clarifying these 😊.. The scripts in the MG5aMC make more sense to me now.
And i'd like to ask if we'd be simulating neutron particles as well? or proton particles are the only interesting beasts of concern?
I'll sure go through the links you shared. Thanks for taking your time to comment on this :)
Neutrons are neutral, so that we cannot use electromagnetic fields to accelerate them. This is the reason why we only accelerate and collide charged particles (protons, antiprotons, electrons, positrons). Here, the reason is thus only a practical one.
Cool.. that makes sense.. So do we get to simulate these other charged particles as well in the #citizenscience project?
Nope, we will only focus on collisions of protons, because this is what the collider in which we are interested (CERN's Large Hadron Collider) does. Other considered options (electron-positron, muon-antimuon or heavy ions) will not be considered here. This would indeed consist in a totally different project, and we cannot ride all horses at the same time ;)
Thanks for your contribution to the STEMsocial community. Feel free to join us on discord to get to know the rest of us!
Please consider delegating to the @stemsocial account (85% of the curation rewards are returned).
You may also include @stemsocial as a beneficiary of the rewards of this post to get a stronger support.